Black Holes and Relativity Discussion
|
|
Watsisname | Date: Monday, 13.06.2016, 09:59 | Message # 16 |
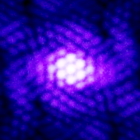 Galaxy Architect
Group: Global Moderators
United States
Messages: 2613
Status: Offline
| Yeah, the simplest, most direct and functional definition of a sphere is that it is the locus of points equidistant from a particular point. This may be in any number of dimensions (circle, sphere, hypersphere, etc.)
If the geometry is Euclidean, then a calculation for the volume enclosed by the sphere is fairly straightforward. You can derive volume formulas by integration.
In curved space, then the Euclidean formulas definitely fail. Area of a circle on the surface of the Earth is definitely not pi*r2. The areas and volumes can still be calculated, it's just more complicated.
This totally changes when we move to space-time. This is not because the space-time is 4D (we can calculate volumes in 4D or in any number of dimensions just fine), but because space-time is not a 4D Euclidean space, even if it is flat. It is not 3D space with another spatial dimension. It is 3D space with a time dimension, so the geometry is Minkowskian, not Euclidean.
Now add curvature to Minkowski space, and try computing volume for a black hole. Immediately we run into a problem. Inside the horizon, the inward radial coordinate becomes a time coordinate. The curvature of the space-time rotates light cones so that the direction towards the singularity becomes the direction of future time.
What.
In computing 3D spatial volume in 4D space-time, the obvious definition for the volume enclosed is with a 3D slice of constant time. But what is a slice with constant time with space-time warped in this way? There is no unique answer -- you have multiple choices. This is why there is no unique answer for the volume enclosed. We can even make the volume enclosed come out to zero!
It may seem very strange that although we can't find a unique result for the volume, we do find a unique result for the surface area. Since the horizon is a null surface, if we choose a different definition for the constant-time space, all we do is shift points in a null direction. Such shifts have zero length and do not change the resulting area.
|
|
| |
midtskogen | Date: Monday, 13.06.2016, 11:28 | Message # 17 |
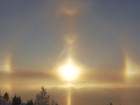 Star Engineer
Group: Users
Norway
Messages: 1674
Status: Offline
| Quote Watsisname (  ) Immediately we run into a problem. Inside the horizon, the inward radial coordinate becomes a time coordinate. The curvature of the space-time rotates light cones so that the direction towards the singularity becomes the direction of future time. So... Let's measure the volume in years from now? :) What happens if we calculate the volume outside the horizon instead?
NIL DIFFICILE VOLENTI
Edited by midtskogen - Monday, 13.06.2016, 11:32 |
|
| |
parameciumkid | Date: Tuesday, 14.06.2016, 00:46 | Message # 18 |
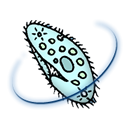 Explorer
Group: Users
United States
Messages: 277
Status: Offline
| I don't have much to add right at the moment, but I'd like to set a few simple things straight while I'm here:
- "an object can never reach the center:" Incorrect. From the perspective of an observer outside the black hole, stationary relative to the black hole, an inertial object is never seen to cross the event horizon. However, the experience of an inertially falling object can be calculated just as easily, and barring unknown laws of physics in the black hole's interior, it actually will reach the center, not only in a finite period of time but quite quickly for the average black hole. The radius, even when calculated in warped coordinates, is a finite and rather small number, and as the object falls faster, it experiences time more slowly and thus will perceive its own trip to the center to be all the shorter. The radius (and thus volume) in "years from now" is really only going to be a few minutes at best.
- An external observer will never see an inertial object enter the event horizon: Sure, but you'll see it get pretty darned close pretty darned quickly. Severe time dilation effects take hold only when its speed becomes close to that of light, i.e. when it's very close to the event horizon. On the scales present in typical real-world black holes, the object will appear to be practically smacking up against it before it slows down all that much. Also note that the slowing down one perceives is a relativistic effect that marches along right in step with several other optical effects, notably dimming, redshifting, and apparent flattening of the object. It won't look like your poor friend slides up to the edge and then just hovers there ethereally. In reality, he'll appear to turn red, then fade to black, all the while flattening until he becomes a nearly two-dimensional silhouette pressed up against the "surface" of the black hole and nearly indistinguishable from it. Ultimately as he appears to get infinitely close, he'll seem to merge with the surface and become one with the black hole.
- Black holes do not explode in supernovae. The death of a black hole due to Hawking radiation is much more of a peaceful withering away. Only when it starts to become extremely small does it start to get hot and decay rapidly, at which point it has a low remaining mass and thus not a lot of energy to release in an explosion. Further, Hawking radiation does not originate inside the black hole, but rather just outside the event horizon. While it does sap mass, the best predictions we've been able to make give no indication that it recovers any information about what had fallen in. In practical terms, throwing an astronaut in and then waiting for an astronaut's mass of Hawking radiation to come back isn't going to result in the astronaut coming back in any form. And while time dilation would appear to make the universe's future history pass you by while you're inside, keep in mind that the time you spend inside is going to be very short from your perspective anyway, and as the time dilation gets worse, the future history will flash by exponentially faster - ultimately all the light you'd be seeing from outside would take the form of an instantaneous pulse of infinitely energetic cosmic rays. Far from escaping, you'd have the more immediate concern of being obliterated right then and there.
Sorry for all the bubble bursting, but that's the rub. The challenge falls to us to come up with even more profound ideas
Intel HD Graphics 4000 ;P
|
|
| |
Watsisname | Date: Tuesday, 14.06.2016, 08:57 | Message # 19 |
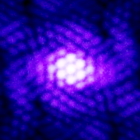 Galaxy Architect
Group: Global Moderators
United States
Messages: 2613
Status: Offline
| Quote expando (  ) People say that once you enter the event horizon you can not escape, I'm not sure is true.
It absolutely is true.
Quote expando (  ) With Hawking Radiation once you enter the event horizon or possibly even before it, time would reach infinity and then the black hole and event horizon will shrink instantly (from the travellers perspective) and then explode in a supernova.
Nope. Paramecium is correct on this.
You fall through the horizon in finite time. And it is short. For a stellar-mass black hole, it's a tiny fraction of a second. In that time, the mass loss of the hole by Hawking radiation is absolutely negligible. (Besides, any black hole large enough to fall into is actually gaining mass more quickly by absorbing CMB photons than losing it by Hawking radiation. But let's ignore that.) The timescale for a solar-mass black hole to evaporate is on the order of 1067 years.
Maybe you point out that things are different for the external observer. Indeed, in the external frame, you never cross the horizon. You only asymptotically approach it. (You still vanish from their view due to redshift, and this happens on the same scale as the in-fall time -- i.e. very quickly.) Suppose they watch you fall toward the hole, and stick around for near eternity and wait for the hole to evaporate. Surely, since you never cross the horizon, and the horizon shrinks and eventually vanishes, they will at some point see you emerge, right?
Wrong. Well, mostly.
What emerges is not you. What emerges is information about you, in the form of scrambled radiation.
From the external perspective, you are thermalized by the environment near the event horizon. As in completely cooked and converted to radiation. Not visibly so to the external viewer, but unavoidably so because the Hawking radiation from that frame of reference is absolutely lethal.
Your own story is different. You experience no such lethal radiation environment (at least not by Hawking radiation). You pass through the horizon, very quickly. You do not experience time going off to positive infinity. You do not witness the black hole's vanishing away by evaporation. You experience destruction by tidal forces and the nasty thing that lies at the center.
Parameciumkid: Everything you said is right. However there is one small distinction to make here:
Quote parameciumkid (  ) And while time dilation would appear to make the universe's future history pass you by while you're inside, keep in mind that the time you spend inside is going to be very short from your perspective anyway, and as the time dilation gets worse, the future history will flash by exponentially faster - ultimately all the light you'd be seeing from outside would take the form of an instantaneous pulse of infinitely energetic cosmic rays. Far from escaping, you'd have the more immediate concern of being obliterated right then and there.
This is untrue for a Schwarzschild black hole. It is (maybe) what happens inside a rotating (Kerr) black hole, and is known as the mass-inflation instability. A fancy way of saying that because the Kerr singularity is gravitationally repulsive, matter piles up near the inner horizon and relative velocities increase without bound. But... this is all dependent on the interior geometry, and we think the Kerr solution is unphysical there.
I'm sure whatever happens there, it's probably pretty lethal. :)
Quote midtskogen (  ) So... Let's measure the volume in years from now? :)
Lol! Okay, how would you do so? What metric component would you be setting to zero?
I think you might misunderstand the problem. The rotated light cones mean that in Schwarzschild coordinates, the interior volume is zero because no constant-time slicings penetrate the horizon. (For coordinates built to describe the black hole's exterior, there is no interior!) But we can choose a different coordinate system to describe the infalling frame, where constant-time slicings do contribute to volume within the horizon. But then the result depends on the choice of the slicing. There is no unique answer.
Quote midtskogen (  ) What happens if we calculate the volume outside the horizon instead?
Same problem. Result depends on your choices!
There is a general lesson here: There is no invariant meaning of 3-volume in space-time. Instead, there is an invariant meaning of 4-volume. Instead of thinking of 3-volumes enclosed by space-like surfaces, you can think of 4-volumes enclosed by a set of world-lines. The black hole, from its conception to evaporation, fills a volume of space-time. That is, it encloses a particular set of events, which are removed from the external universe by a horizon. That set of events is the black hole. What is its 4-volume? Depends on its history!
|
|
| |